The FERMI space telescope celebrates its 15th anniversary: 15 years observing the gamma rays of the Universe … and detecting their sources!
15 years ago, NASA launched the Fermi space mission, equipped with a telescope capable of observing the gamma rays of the universe, at energies several billion to several hundred billion times that of visible light. The primary objective of this mission was to explore the origin and life of cosmic radiation, a population of relativistic particles that bathe galaxies and witness the most violent and extreme phenomena in our cosmos, such as novae, supernovae, pulsars and supermassive black holes. This phenomenon, which has been known for over a century, still holds a great deal of mystery, but it can also tell us a great deal about the astrophysical objects that give rise to it, as well as about some fundamental physical processes, such as plasma dynamics.
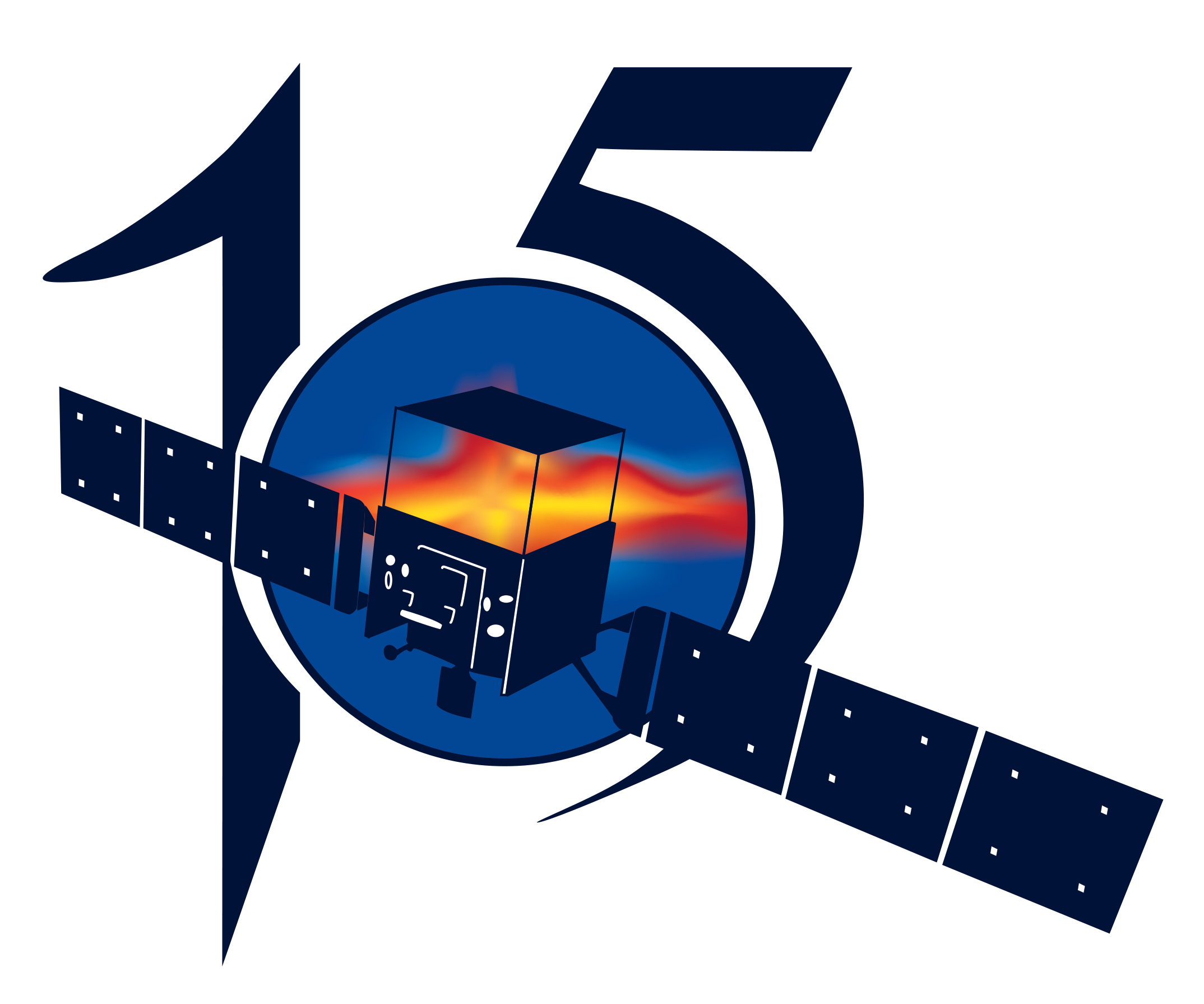
IRAP has been part of this adventure from before the launch to the present day, and this anniversary gives us the opportunity to look back at some of our team’s work, within IRAP’s GAHEC group and in collaboration with numerous French and foreign students and colleagues. The quality of the continuous observations provided by the mission’s main instrument, the Large Area Telescope (LAT), has enabled us to take an interest over the years in almost all the richness of what Fermi gives us to see of our Universe in its most energetic manifestations. Here’s a look back at a selection of our discoveries:
The richness of the gamma-ray sky
Thousands of particle boosters are at work in the Universe, according to 15 years of Fermi observations (1). Cataloguing all these objects and associating them with known stars has been the task of IRAP researchers, in order to bring some order to this abundance. The first category of objects, with several thousand representatives, are blazars, supermassive black holes at the center of galaxies, which accelerate a jet of matter towards the Earth. The second category of objects, comprising around a third of sources, includes emitters that are not associated with any known object, stimulating observations at other wavelengths to understand their nature. Next in line are pulsars, neutron stars whose pulsed light beams testify to their rapid rotation. Then there are other, rarer objects, such as supernova remnants, globular clusters, binary stars or nearby galaxies, which are part of those special environments capable of accelerating matter to near-light speeds.
Unexpected boosters
In 2010, the LAT detected an unexpected gamma-ray emission lasting around ten days, coming from a nova, V407 Cyg, located in our Galaxy 2.7 kpc from Earth (1). Since then, Fermi has observed gamma-ray emission from one nova every year or so, confirming that they are a new class of high-energy photon source (2,3). The nova phenomenon is explained by a thermonuclear explosion on the surface of a white dwarf, which begins to glow hundreds of thousands of times brighter than the Sun. The explosion ejects the dwarf’s envelope at thousands of km/s. The collision of the high-velocity ejected gas with the expanding dwarf envelope or the wind from the companion star accelerates protons to relativistic velocities, and these produce gamma-ray emission as they interact with the surrounding matter (4). The energy dissipated in these shocks helps to make the novae glow. These sources are therefore a veritable laboratory for studying particle acceleration phenomena, and their gamma-ray emission provides a better understanding of the origin of novae luminosity in the visible range.
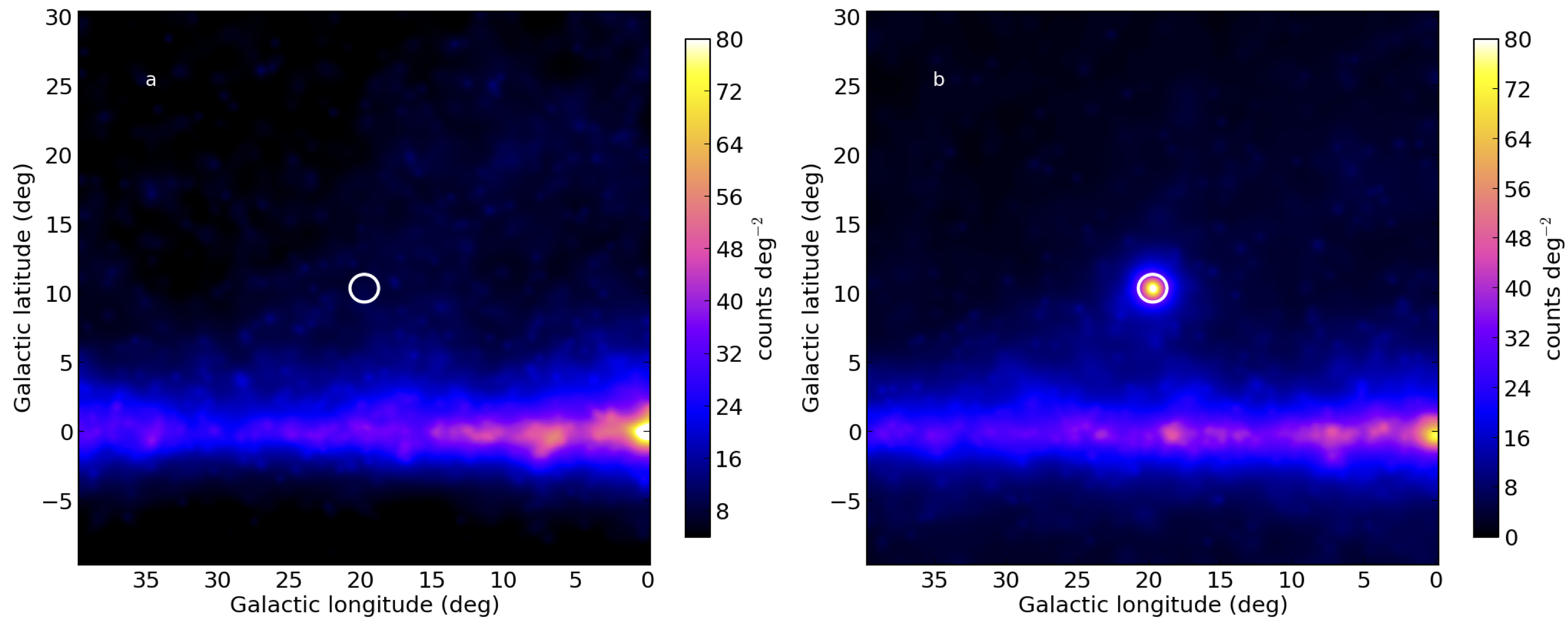
An extraordinary neighbor
The Large Magellanic Cloud is a dwarf galaxy orbiting the Milky Way. Its proximity gives us an unparalleled view of a galaxy from the outside. LAT observations analyzed at IRAP have revealed that cosmic radiation seems to propagate in this galaxy in a different way to that which prevails in the Milky Way, making this object an excellent test of our theories on cosmic radiation (1). On the other hand, the Large Magellanic Cloud is home to some extraordinary emitters: the most powerful gamma-ray pulsar known (2), a massive star-compact object binary system that is the most luminous in its class (3), etc. All these extraordinary sources will help us to better understand the mechanisms responsible for particle acceleration in the Universe.
At the source of extreme energies
Cosmic radiation from our Galaxy reaches extreme energies per particle, and the process by which this happens remains a mystery. The most prolific particle boosters, such as supernovae, don’t seem to be able to accomplish this feat. For some twenty years now, star-forming regions have been suspected of playing an important role in the ecology of cosmic radiation, due to the concentration of power they represent. The IRAP team focused on the Cygnus region, a unique concentration of massive stars in the solar neighbourhood, and showed that the high-energy gamma-ray emission from this region could be explained by a single past supernova as much as by a process of continuous acceleration by the stellar clusters found there (1). So the mystery remains, and the question requires a new approach!
Anti-stars in the Galaxy?
The laws of physics tell us that the Universe should contain equal amounts of matter and antimatter. Yet antimatter is only observed in tiny quantities. Recently, the AMS-02 particle detector on board the International Space Station seems to indicate that there may be more antimatter around us than previously thought. This could be in the form of stars made of antimatter, or anti-stars (1). We know that the collision between antimatter and matter produces gamma rays. Researchers at IRAP have excavated ten years of Fermi data and found fourteen gamma-ray sources whose emission properties are comparable to those expected from antistars. However, the nature of these sources is still uncertain. The IRAP team estimated the maximum number of anti-stars that could exist in our Galaxy, obtaining the strongest constraints ever established: in the galactic disk there is less than one anti-star for every 300,000 ordinary stars (2).
The Fermi mission is still fully operational, and the international scientific community hopes to see its gamma-ray eye roaming the skies for many years to come. With the advent of multi-messenger astronomy (neutrinos, ultra-high-energy cosmic rays, gravitational waves) and growing interest in transient astrophysical phenomena (gamma-ray bursts, fast radio bursts, mergers of compact objects), a mission that scans almost the entire sky in just a few hours is a considerable asset. In addition, the advances in our understanding of the sky at high energies made possible by Fermi play a key role in the definition of future instruments in the discipline, such as the Cherenkov Telescope Array.
Further Resources
Scientific papers :
- Catalog:
- (1) S. Abdollahi, F. Acero, L. Baldini, J. Ballet, D. Bastieri, et al., The Astrophysical Journal Supplement Series, 260, 53, 2022
- Novae:
- (1) Abdo et al., Science, 329, 817, 2010
- (2) Ackermann et al., Science, 345, 554, 2014
- (3) C.C. Cheung et al., The Astrophysical Journal, 826, 142, 2016
- (4) P. Martin, G. Dubus, P. Jean, V. Tatischeff & C. Dosne, Astronomy and Astrophysics, 612, A38, 2018
- Large Magellanic Cloud:
- (1) M. Ackermann, A. Albert, W.B. Atwood, L. Baldini, J. Ballet, et al., Astronomy and Astrophysics, 586, A71, 2016
- (2) M. Ackermann, A. Albert, L. Baldini, J. Ballet, et al., Science, 350, 801, 2015
- (3) R.H.D. Corbet, L. Chomiuk, M.J. Coe, J.B. Coley, G. Dubus, et al., The Astrophysical Journal, 829, 105, 2016
- Stellar clusters:
- (1) X. Astiasarain, L. Tibaldo, P. Martin, J. Knödlseder, Q. Rémy, Astronomy and Astrophysics, 671, A47, 2023
- Anti-stars:
- (1) V. Poulin, P. Salati, I. Cholis, M. Kamionkowski, J. Silk, Physical Review D, 99 2 023016, 2019
- (2) S. Dupourqué, L. Tibaldo, P. von Ballmoos, Physical Review D, 103 083016, 2021
IRAP Contacts
- Pierrick Martin, pierrick.martin@irap.omp.eu
- Luigi Tibaldo, luigi.tibaldo@irap.omp.eu
- Pierre Jean, pjean@irap.omp.eu
- Jürgen Knödlseder, jurgen.knodlseder@irap.omp.eu
- Peter von Ballmoos, Peter.VonBallmoos@irap.omp.eu